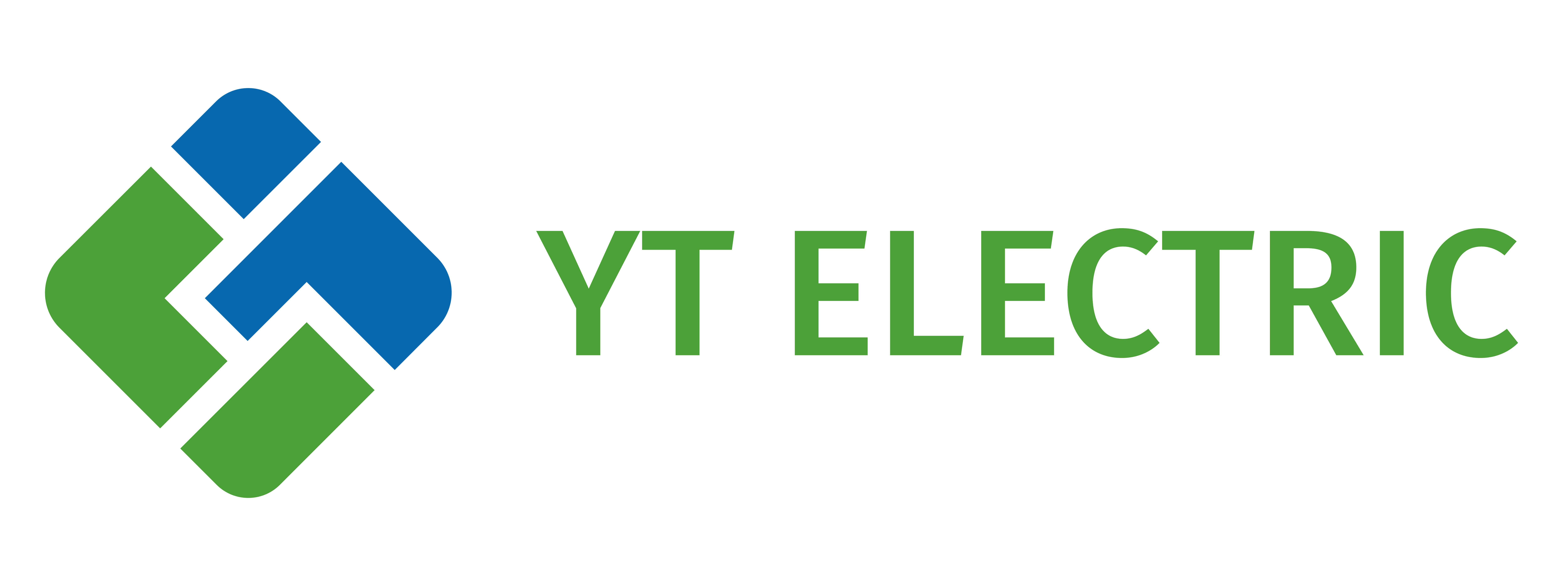
Capacitors are important parts of electronic circuits. They play key roles in power supply systems, signal processing, and energy storage. Their electrical properties directly affect circuit performance, efficiency, and reliability. Engineers and circuit designers must understand these properties to ensure optimized design and system stability.
This article looks at the main electrical features of capacitors. These include capacitance, leakage current, and equivalent series resistance (ESR). It also covers dielectric loss, self-resonant frequency (SRF), voltage rating, and temperature coefficient. Additionally, it discusses parasitic effects and more. By delving into these properties, designers can better select capacitors suited for specific applications and optimize overall circuit performance.
1. Capacitance: The Core Attribute of a Capacitor
Capacitance is the key electrical property of a capacitor. It shows how well a capacitor can store electrical charge. This charge is stored between two conductive plates. These plates are separated by a dielectric material. It is measured in farads (F), and its value depends on the geometry of the capacitor and the properties of the dielectric. The capacitance formula is:
Where:
C = capacitance
ε = permittivity of the dielectric material
A = area of the plates
d = distance between the plates
The larger the capacitance, the more charge a capacitor can store. This is important for energy storage, filtering, and smoothing voltage. In real-world circuit design, choosing the right capacitance is not just about storage needs. You also need to think about frequency response, impedance matching, and physical size.
In high-frequency circuits, smaller capacitors are often favored because they can handle fast-changing signals effectively. In contrast, large capacitance values are essential in low-frequency applications like power supply ripple filtering, ensuring a smooth output voltage.
2. Leakage Current: The Unwanted Loss of Charge
Leakage current is a small amount of current that flows through the dielectric material of a charged capacitor. This causes the stored energy to slowly discharge over time. Ideally, a capacitor should hold its charge indefinitely, but in practice, imperfections in the dielectric lead to leakage. The amount of leakage current directly affects the performance of a capacitor, particularly in energy storage and timing-sensitive applications.
For example, in low-power circuits or energy harvesting systems, excessive leakage current can lead to premature discharge, reducing the overall efficiency or accuracy of the system. Electrolytic capacitors usually have higher leakage currents. In contrast, ceramic and film capacitors have lower leakage currents. This makes ceramic and film capacitors better for applications that need long-term charge retention.
In circuits that require long-term stability, such as in timing circuits or memory devices, the ability to retain charge for extended periods without significant leakage is essential for accurate performance.
3. Equivalent Series Resistance (ESR): Hidden Energy Losses
Equivalent Series Resistance (ESR) represents the internal resistance of a capacitor and is an essential parameter, especially in high-frequency and high-power applications. ESR accounts for resistive losses within the capacitor, including contributions from the leads, plates, and dielectric. In an ideal capacitor, ESR would be zero, but in reality, it can affect the overall performance of the capacitor.
In alternating current (AC) circuits, the energy loss due to ESR can be significant, particularly in high-frequency applications. The power dissipation resulting from ESR can be calculated as:
Where Ploss is the power loss, III is the current through the capacitor, and ESRESRESR is the equivalent series resistance. High ESR leads to increased power dissipation in the form of heat, which can negatively impact efficiency and, over time, cause thermal damage to the capacitor.
In applications such as switch-mode power supplies (SMPS) and RF circuits, high ESR can degrade performance by introducing excess voltage ripple and noise, reducing energy efficiency, and generating unnecessary heat. Low-ESR capacitors, like ceramic or high-quality film capacitors, are preferred in these environments for their ability to minimize energy loss and enhance system performance.
4. Dielectric Loss: Energy Dissipation in the Dielectric
Dielectric loss occurs when the dielectric material inside a capacitor absorbs energy from an alternating electric field and converts it into heat. This energy dissipation is critical in AC applications, especially at higher frequencies, as it reduces the overall efficiency of the capacitor.
The dissipation factor (DF) or loss tangent (tanδ) is a key metric used to quantify dielectric loss:P_{\text{loss}} = I^2 \cdot \text{ESR}
Where δ is the loss angle, Rleak is the leakage resistance, and XC is the capacitive reactance. A smaller dissipation factor indicates lower energy losses and greater efficiency, which is particularly important in signal filtering, high-frequency applications, and precision instrumentation.
For example, in RF circuits, capacitors with high dielectric loss can attenuate or distort signals. To maintain signal integrity and minimize energy waste, it is essential to choose capacitors with low-loss dielectrics, such as mica or specific ceramic types, when working with high-frequency circuits.
5. Self-Resonant Frequency (SRF): The Frequency Limit of a Capacitor
Self-Resonant Frequency (SRF) refers to the frequency at which a capacitor no longer behaves purely as a capacitive element and instead begins to exhibit inductive properties due to parasitic inductance. SRF is especially important in high-frequency and RF applications, where capacitors are used for filtering, signal decoupling, and impedance matching.
At the self-resonant frequency, the inductance and capacitance of the capacitor form a resonant circuit, and beyond this point, the capacitor acts more like an inductor. The SRF can be calculated using the formula:
Where LLL represents the parasitic inductance, and CCC is the capacitance. Capacitors with lower parasitic inductance exhibit higher SRF, making them suitable for high-frequency applications. When selecting capacitors for RF circuits, designers must ensure that the capacitor’s SRF is well above the circuit’s operating frequency to prevent performance degradation.
In high-frequency applications, such as RF signal filtering and impedance matching networks, the SRF of a capacitor is crucial. If the operating frequency gets close to or goes above the SRF, the capacitor's performance drops. This affects the circuit's stability and efficiency.
6. Voltage Rating: Ensuring Safe Operation
The voltage rating of a capacitor indicates the maximum continuous voltage that it can withstand without experiencing dielectric breakdown. If the applied voltage exceeds the rated voltage, the capacitor may suffer permanent damage, including dielectric breakdown or a short circuit.
Capacitors are rated based on the dielectric material and construction. Electrolytic capacitors, for instance, typically have lower voltage ratings compared to ceramic or film capacitors. For safety and longevity, it is advisable to operate capacitors at no more than 70%-80% of their maximum rated voltage to avoid breakdown.
In applications such as high-voltage power supplies or energy storage systems, selecting a capacitor with a sufficiently high voltage rating is critical to prevent premature failure due to voltage spikes or transient events. By ensuring that the operating voltage is within safe limits, designers can avoid catastrophic failures and enhance the reliability of their systems.
7. Temperature Coefficient: Stability Over Temperature
The temperature coefficient of a capacitor describes how its capacitance value changes with variations in temperature. Capacitors with positive temperature coefficients will see their capacitance increase as the temperature rises, while capacitors with negative coefficients will experience a decrease in capacitance as the temperature increases.
In precision circuits, such as oscillators or timing circuits, maintaining a stable capacitance value over a range of temperatures is critical. A high temperature coefficient can lead to unwanted capacitance changes, causing frequency drift or inaccuracies in timing applications. Capacitors with low temperature coefficients, such as NP0 (C0G) ceramic capacitors, are ideal for temperature-sensitive applications.
Furthermore, operating temperatures can degrade the dielectric material inside capacitors, especially at extreme temperatures. Capacitors used in harsh environments, such as automotive or industrial settings, must have a stable temperature coefficient and be designed to withstand high temperatures without experiencing significant performance degradation.
8. Parasitic Effects: Inductance and Resistance in Real Capacitors
In addition to ESR and dielectric losses, real-world capacitors also exhibit parasitic inductance and resistance, which can impact performance, particularly in high-frequency applications. These parasitic components arise from the physical construction of the capacitor, including the leads, terminals, and internal geometry.
Parasitic inductance becomes significant at high frequencies, where it can interfere with the intended capacitive behavior. Instead of acting purely as a capacitor, the component may exhibit inductive properties, leading to oscillations, signal distortions, or reduced filtering performance.
To mitigate parasitic effects, designers can use surface-mount capacitors (which have lower lead inductance) or select capacitors specifically designed for high-frequency applications, such as ceramic chip capacitors. Additionally, optimizing PCB layouts and minimizing the length of capacitor leads can help reduce parasitic inductance and improve circuit performance.
9. ESR in High-Power Applications: Heat and Efficiency Concerns
In high-power applications such as inverters or power converters, the equivalent series resistance (ESR) of a capacitor plays a critical role in determining efficiency and thermal performance. As mentioned earlier, ESR contributes to power loss in the form of heat dissipation. In high-current environments, this heat can accumulate rapidly, leading to increased operating temperatures and potentially damaging the capacitor.
In power electronics, selecting capacitors with low ESR is essential to ensure efficient energy transfer and to minimize the risk of overheating. For example, film capacitors and ceramic capacitors with low ESR are commonly used in power supply circuits to filter out noise and maintain voltage stability.
10. Selecting the Right Capacitor for the Application
Choosing the right capacitor for a specific application requires a thorough understanding of the circuit’s requirements and the electrical properties of available capacitors. Key factors to consider include capacitance, voltage rating, ESR, dielectric loss, SRF, and temperature stability.
For example:
Power supply filtering: Requires capacitors with large capacitance values and low ESR to handle high currents and maintain voltage stability.
High-frequency RF applications: Require capacitors with low parasitic inductance, high SRF, and minimal dielectric loss to preserve signal integrity.
Precision timing circuits: Need capacitors with stable temperature coefficients and minimal leakage current to ensure consistent performance over time.
By matching the capacitor’s properties with the demands of the application, designers can maximize efficiency, reliability, and overall system performance.
Conclusion
Capacitors exhibit a range of complex electrical properties that significantly impact their behavior in various applications. From basic capacitance and leakage current to more advanced parameters like ESR, dielectric loss, SRF, and temperature coefficient, each characteristic plays a crucial role in determining the capacitor’s performance under different conditions.
Understanding these properties allows engineers to select the right capacitor for specific applications, whether for filtering, decoupling, energy storage, or signal processing. By carefully considering capacitance, ESR, voltage rating, temperature stability, and other factors, capacitors can be optimized to enhance circuit performance, increase efficiency, and ensure long-term reliability in even the most demanding environments.
As capacitor technologies continue to evolve, new materials and designs will further improve their electrical properties, enabling more efficient and high-performance electronic systems for the future. In particular, the integration of Static Var Generators (SVG) and Active Harmonic Filters (AHF) into power systems represents a significant advancement. These devices offer superior performance in power quality management, with SVGs providing dynamic reactive power compensation and AHFs effectively mitigating harmonic distortions. This combination ensures stable and clean power supply, making them essential components for modern industrial and commercial applications that demand high reliability and efficiency.
Subscribe to us to enjoy event prices and get some of the best prices.